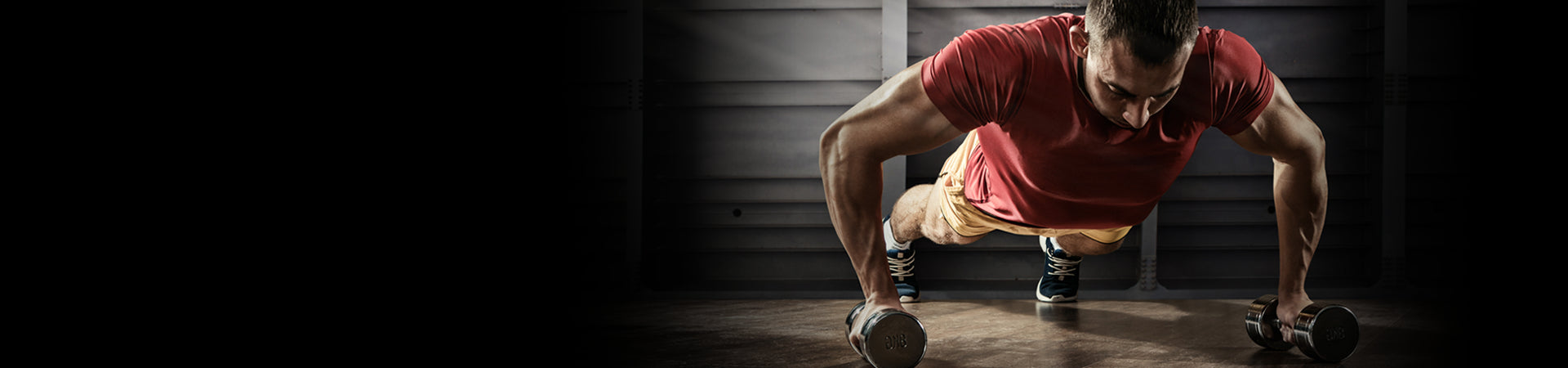
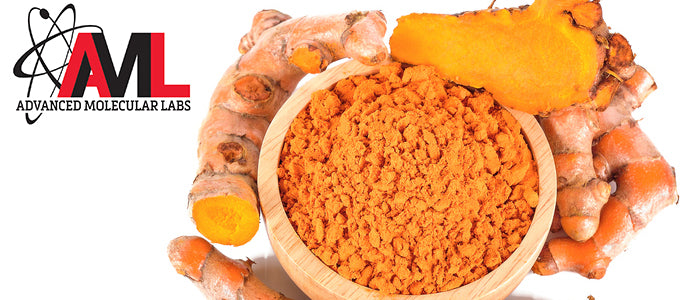
Curcumin: Testosterone Booster and Fat Burner
Curcumin is a naturally occurring polyphenolic compound derived from the turmeric plant. It is commonly used in many parts of the world as a spice and medical agent to treat a wide variety of ailments.1 Research has identified curcumin as the active compound responsible for most of the health benefits associated with turmeric consumption2, which include anti-inflammatory, anti-cancer and antioxidant effects.3,4 The wide-ranging biological activity of curcumin comes from its versatile molecular structure that provides the unique capacity to bind and regulate numerous biological targets. So what is the relationship between curcumin and testosterone? Well, in addition to the many targets that curcumin regulates to improve overall health, curcumin also affects additional biomolecules— resulting in greater production of the muscle-building hormone testosterone. Curcumin also triggers the remarkably powerful fat-reducing process known as thermogenesis.
Powerful Antioxidant Capacity
Normal cellular function involves metabolic processes that generate oxidative stress on the cell. Research has found that increased oxidative stress within certain testicular cells, known as Leydig cells, can lower testosterone production.5 In addition, scientists have also found that certain antioxidants, such as vitamin E, mitigate oxidative stress which slows down the age-related decline of testosterone production.6 These results suggest that consumption of more powerful antioxidants, such as curcumin, may decrease oxidative stress enough to actually increase testosterone levels.
In order to see if this was possible, a group of Nigerian scientists investigated whether consumption of curcumin increased testosterone.7 In this study, four groups of young male rats were given gallic acid (which acts as a pro-oxidant and reduces testosterone), curcumin, curcumin plus gallic acid or a placebo. At the end of the study, the researchers noted the following:
• The testosterone concentration of the gallic acid-consuming group was roughly 30 percent lower than in the control group receiving the placebo. The gallic acid group also demonstrated considerable oxidative stress based on the significant drop seen in endogenous antioxidant molecules within the testes, because they were expended while scavenging molecules, causing oxidative stress.
• In the group ingesting curcumin, testosterone concentration was 260 percent higher than in the control group, demonstrating that curcumin can stimulate testosterone production.
• The group ingesting gallic acid and curcumin also showed an increase in testosterone of 180 percent, establishing curcumin’s capacity to overcome the oxidative stress induced by gallic acid and still increase testosterone production.
• Interestingly, both groups consuming curcumin showed less oxidative stress based on their normal levels of endogenous antioxidants. Furthermore, the raised testosterone level found in the curcumin-consuming groups was, in part, the consequence of increased activity of two key enzymes involved in the biosynthesis of testosterone known as 3 beta-HSD and 17 beta-HSD.
The researchers concluded that curcumin reduced oxidative stress within the testes, promoting better testicular function— resulting in enhanced testosterone production.
Decreased Estrogen Production
In addition to curcumin directly increasing testosterone, it also may increase testosterone in a more indirect way based on a recent study by Zhang et al.8 showing that curcumin reduced estrogen production. While all of the molecular details regarding curcumin’s ability to decrease estrogen are unknown, this lower amount of estrogen should boost testosterone levels by acting as a signal to the brain that there is a lack of circulating steroid hormones in the blood. As a result, the brain will react by increasing luteinizing hormone (LH) secretion from the anterior pituitary gland, which stimulates testicular production of testosterone.
Activating the Muscle-Enhancing Vitamin D Receptor
Unlike many other vitamins, vitamin D has the unique ability to directly regulate genetic expression.9 It does this by binding and activating the vitamin D receptor, which subsequently interacts with specific DNA regulatory elements that switch gene expression on or off. More specifically, vitamin D has been shown to regulate the expression of several genes involved in the production of testosterone.10 Interestingly, a recent study by Bartik et al.11 demonstrated that curcumin can also bind to the vitamin D receptor and turn on the expression of several genes. Although none of the genes turned on by curcumin in this study were testosterone-producing genes, this study did not exhaustively investigate the expression of the many genes influenced by the activated vitamin D receptor— allowing for the good possibility that curcumin does, in fact, activate genes involved in testosterone biosynthesis. This is even more probable after taking into consideration the previously shown capacity of curcumin by Abarikwu et al.7 to effectively increase testosterone production.
Curcumin Uniquely Ignites Thermogenesis
Two essential requirements to effectively promote fat loss are increased fatty acid oxidation and energy expenditure. Curcumin stimulates both of these fat-eliminating necessities by promoting thermogenesis, which uncouples the normally linked process of fat burning with cellular energy (ATP) production within mitochondria. As a result, instead of the energy from fat being used to synthesize ATP, it is instead converted into heat, which effectively increases energy expenditure. In addition, curcumin’s energy uncoupling capacity significantly decreases ATP biosynthesis, signaling a low cellular energy state that activates the cell’s master energy regulator, AMPK. Upon activation, AMPK recharges the cell’s energy status by cranking up energy-producing processes such as fatty acid oxidation, which therefore achieves the second essential requirement for effective fat loss.
Brown adipose tissue (BAT) has been shown to be the most proficient thermogenic tissue in the body because of its relatively high number of mitochondria that are loaded with the uncoupling protein 1 (UCP-1) which, as its name implies, is the protein that directly uncouples fat oxidation with ATP production and drives thermogenesis. However, since all cells have mitochondria, they do possess the ability to support thermogenesis. In fact, a study by Lim et al.12 showed that curcumin can actually uncouple fat burning with energy production, eliciting thermogenesis within mitochondria from liver cells. Interestingly, curcumin activates thermogenesis by functioning as an energy-uncoupler itself, which alleviates the requirement for UCP-1. This may be the reason that curcumin can activate thermogenesis in non-BAT tissue that do not possess significant amounts of UCP-1.
In closing, the newly released fat-scorching product Thermo Heat has an exclusive blend of curcumin-like compounds, known as curcuminoids, which conceivably attack body fat and increase testosterone production in a similar manner to curcumin— and thus provide the same remarkable ability to boost fat loss while enhancing muscle hypertrophy.
For most of Michael Rudolph’s career he has been engrossed in the exercise world as either an athlete (he played college football at Hofstra University), personal trainer or as a Research Scientist (he earned a B.Sc. in Exercise Science at Hofstra University and a Ph.D. in Biochemistry and Molecular Biology from Stony Brook University). After earning his Ph.D., Michael investigated the molecular biology of exercise as a fellow at Harvard Medical School and Columbia University for over eight years. That research contributed seminally to understanding the function of the incredibly important cellular energy sensor AMPK— leading to numerous publications in peer-reviewed journals including the journal Nature. Michael is currently a scientist working at the New York Structural Biology Center doing contract work for the Department of Defense on a project involving national security.
References:
1. Sharma RA, Gescher AJ and Steward WP. Curcumin: the story so far. Eur J Cancer 2005;41, 1955-1968.
2. Aggarwal BB, Sundaram C, et al. Curcumin: the Indian solid gold. Adv Exp Med Biol 2007;595, 1-75.
3. Abe Y, Hashimoto S and Horie T. Curcumin inhibition of inflammatory cytokine production by human peripheral blood monocytes and alveolar macrophages. Pharmacol Res 1999;39, 41-47.
4. Kawanishi S, Oikawa S and Murata M. Evaluation for safety of antioxidant chemopreventive agents. Antioxid Redox Signal 2005;7, 1728-1739.
5. Diemer T, Allen JA, et al. Reactive oxygen disrupts mitochondria in MA-10 tumor Leydig cells and inhibits steroidogenic acute regulatory (StAR) protein and steroidogenesis. Endocrinology 2003;144, 2882-2891.
6. Cao L, Leers-Sucheta S and Azhar S. Aging alters the functional expression of enzymatic and non-enzymatic antioxidant defense systems in testicular rat Leydig cells. J Steroid Biochem Mol Biol 2004;88, 61-67.
7. Abarikwu SO, Akiri OF, et al. Combined administration of curcumin and gallic acid inhibits gallic acid-induced suppression of steroidogenesis, sperm output, antioxidant defenses and inflammatory responsive genes. J Steroid Biochem Mol Biol 2014;143C, 49-60.
8. Zhang Y, Cao H, et al. Curcumin inhibits endometriosis endometrial cells by reducing estradiol production. Iran J Reprod Med 2013;11, 415-422.
9. Ramagopalan SV, Heger A, et al. A ChIP-seq defined genome-wide map of vitamin D receptor binding: associations with disease and evolution. Genome Res 2010;20, 1352-1360.
10. Reichel H, Koeffler HP and Norman AW. The role of the vitamin D endocrine system in health and disease. N Engl J Med 1989;320, 980-991.
11. Bartik L, Whitfield GK, et al. Curcumin: a novel nutritionally derived ligand of the vitamin D receptor with implications for colon cancer chemoprevention. J Nutr Biochem 2010;21, 1153-1161.
12. Lim HW, Lim HY and Wong KP. Uncoupling of oxidative phosphorylation by curcumin: implication of its cellular mechanism of action. Biochem Biophys Res Commun 2009;389, 187-192.